Protecting Investments in Submarine Cables
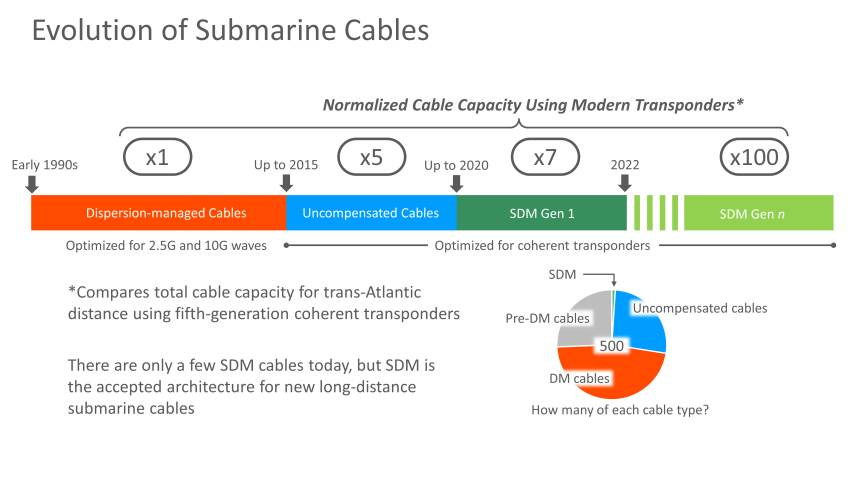
By Geoff Bennett
From time to time at dinner parties I need to explain what I do, and I’m surprised how many people, even within the telecom space, where my specialty lies, think that international traffic passes over satellites (great work by SpaceX marketing!). I politely point out that over 99.5 percent of intercontinental internet traffic passes over submarine cables, a statistic that was confirmed in the Telecom Mythbusters session at the SubOptic conference in Bangkok earlier this year. These cables are also responsible for over $10 trillion in financial transactions per day, and they enable the speed of our social media updates.
A new cable could cost more than $100 million and take years to plan and deploy. Newer generations of cable will typically be able to carry much more capacity than older cables of about the same length. But once a cable has been deployed, the only things we can change to increase capacity are the transponders at the ends of the cable. The rest of the system will stay sitting on the seabed for the rest of its life.
Submarine cable technology has evolved dramatically over the past 30 years. I’ll give an overview in this article, referencing trans-Atlantic cables as a baseline because it’s possible to find cables there of different generations that are about the same length and provide like-for-like comparisons.
Submarine Cable Generations
Long-distance submarine cables can, broadly speaking, be put into three different categories:
- Dispersion-managed cables designed for 2.5 Gb/s and 10 Gb/s direct-detect transmission, which were deployed from the early 1990s onward.
- Analog dispersion management techniques within the cable evolved over time, with at least two distinct approaches, but the focus was always to assume that no dispersion compensation was needed in the transponder and to maximize capacity per fiber pair.
- The first generation of coherent transponders was deployed on this type of cable and delivered a massive performance boost.
- However, these cables were not specifically designed for coherent transmission, and this results in certain characteristics that limit their ultimate performance, even when using the latest transponders. For this reason, I have used them as a baseline of x1 in terms of performance.
- Uncompensated cables designed for coherent transmission, which were deployed from around 2015 onward.
- These cables typically operate at high repeater gain to help enable high-order modulation and high spectral efficiency. They usually use large-effective-area fibers with positive dispersion that accumulates along the whole length of the cable, and thanks to these two properties, nonlinear penalties are minimized. Dispersion is compensated for in the coherent transponder using digital signal processing (DSP). Uncompensated cables also focus on maximizing capacity per fiber pair.
- The MAREA is an example of this type of cable. Note that MAREA delivers the highest performance of any existing trans-Atlantic cable in terms of fiber pair capacity at x5 cable capacity.
- Modern transponders are so successful that they operate within a couple of decibels of the theoretical capacity limit for these cables. For this reason, the next step in cable design moves its focus away from capacity per fiber pair and toward overall cable capacity. This new design philosophy is called space-division multiplexing (SDM).
- Space-division multiplexing cables.
- These cables are also designed for coherent transmission, with DSP dispersion compensation and positive-dispersion fiber, but their design philosophy moves away from maximizing capacity per fiber pair in favor of maximizing the capacity of the cable as a whole. SDM repeaters operate at lower power levels, well within the linear regime, and these cables typically use more traditional and less expensive optical fiber types.
- There is already an evolving roadmap for SDM, with first-generation designs deployed from 2020 and extending to trans-Atlantic-length cables with multi-petabit capacity in the next 10 years.
- The Dunant cable is an example of a first-generation SDM cable, with about x7 performance.
- In several submarine networking conferences, we have seen roadmaps for future SDM evolution with capacity up to the 4 petabit level for a trans-Atlantic cable. It may be possible to push this limit even more, to x200 capability, but there are many new and untested technologies involved in this type of cable.
Coherent Transponders
Coherent transponders were first commercialized around 2009 and are now in their fifth distinct generation, with commercial transponders that can deliver up to 800 Gb/s per wavelength. During this evolution, there were two clear directions of evolution: increasingly sophisticated modulation and higher baud rates.
A coherent transponder sends data using modulation symbols that hold a certain number of data bits. Modulation constellations have become increasingly sophisticated over time, and as this evolution progressed, each symbol was able to carry more bits. A PM-QPSK symbol, for example, carries 4 bits, while a PM-64QAM symbol carries 12 bits. By carrying more bits in each symbol, we increase the spectral efficiency (and thus the fiber capacity) of the system. We’ve seen a threefold increase in spectral efficiency over this period.
Unfortunately, carrying more bits per symbol also has the effect of reducing the optical reach of the transmission, with a 30-fold reduction in reach if we compare PM-QPSK with PM-64QAM. In the latest generation of coherent transponders, we have seen the first effective implementations of a technique called probabilistic constellation shaping (PCS). PCS is quite a complex idea to explain, but the result is fairly simple. It can boost the optical performance of a modulation symbol in two ways:
- It offers a much more granular way to carry the bits compared to conventional constellations, so it’s possible to squeeze out additional signal quality for a given wavelength data rate at a given optical reach.
- By reducing the probabilities of using the highest-power modulation symbols (power increases as you move out from the origin), we can achieve better transmission performance.
Conventional wisdom is that PCS will always deliver the optimum capacity reach.
Increasing Baud Rates
The baud rate is the rate at which we send modulation symbols. For an 800 Gb/s signal that uses a full PM-64QAM constellation (12 bits per symbol), for example, we would need a minimum rate of 84 gigabaud. This allows not only the 800 Gb/s payload, but also the overhead of framing and forward error correction (FEC) to be transmitted. But by increasing the baud rate, it can be possible to increase the optical reach of a given data rate.
For example, an 84 Gbaud PM-64QAM signal with a nominal reach factor of 1 using the full 64QAM constellation with no PCS would yield a very short reach. By increasing the baud rate to 96 Gbaud, a relatively small increase, we see a fourfold increase in reach because we can “dial back” the number of bits per symbol to 10.5 using PCS.
Increasing the baud rate still further to 100 Gbaud delivers a fivefold increase in reach because we’ve dialed back PCS still further to carry only 10 bits per symbol.
Conventional wisdom is to always operate with the highest baud rate to achieve optimum capacity reach.
Why Defy Conventional Wisdom?
Conventional wisdom is extremely compelling in terrestrial networks, where provisioning hundreds of services over multiple routes in a meshed long-distance network must be a “production line” process with minimum manual intervention. Capacity is so precious in a submarine cable that it’s worth the effort to squeeze out every last gigabit per second.
For example, if we were to design a transponder with excellent, granular PCS but perhaps just a “high” and “low” setting for baud rates, we would miss an opportunity for such optimization because we will often find that a given wavelength could support, for example, 626 Gb/s or 642 Gb/s. We may have granularity, but the Ethernet clients that use this wavelength work in chunks of 100 Gb/s or 400 Gb/s. Flexible data rate Ethernet (FlexE) was supposed to address this issue, but it’s not widely implemented, and even if it was, it would be tricky for subsea operators to sell a flexible service.
Turning this signal down to 600 Gb/s and then tuning the baud rate down a little from the maximum rate, we find that the spectral width of the signal will become narrower and, given enough channels across the band, we can squeeze extra channels in to use up the “stranded” capacity. We’ve found that we can extract up to 25 percent more capacity from a modern uncompensated or SDM cable in this way.
Similarly, there are types of cable in which PCS does not always deliver the best result, especially cables that are older and have high nonlinear penalties. PCS loses its mojo at a spectral efficiency of PM-QPSK or lower, and we find it’s better to switch it off and use non-PCS fixed modulations with special characteristics that suit these fiber conditions. In some challenging cables, we’ve extracted up to 50 percent more capacity than a PCS-only implementation.
The Past and Future of Submarine Transponders
Early coherent transponders circa 2008 were designed only for terrestrial transmission, and it was pure luck that they worked so effectively on the submarine cables of that era. Over the last 15 years of coherent development, it has been essential that the same coherent DSP chips can be used by both terrestrial and submarine network markets. The cost of developing the latest DSP technology runs up to $70 million, and this scale of investment could not be recouped if the chip set could only be used in submarine deployments.
Most features from a typical advanced coherent toolkit for a fifth-generation transponder apply to both terrestrial and submarine network deployments, but a few of the features were developed either exclusively for subsea operation or can be used to deliver a significant performance boost for submarine cables. One of the earliest examples of this was for the second and subsequent generations of coherent transponder to be able to compensate for very high levels of chromatic dispersion that are encountered in uncompensated cable designs.
In addition to the variable baud rates and non-PCS modulations, features such as super-Gaussian PCS, SD-FEC gain sharing and high-gain FEC modes are all valuable for certain types of submarine cables. Across a variety of cable types, these features can deliver 15 to 50 percent more capacity compared to a transponder without them.
As we look to future generations of submarine transponders, it’s essential to maintain this link to terrestrial applications while adding subsea-specific features.
Conclusion
Modern coherent transponders have a wide selection of digital tools available to help them extract maximum capacity from submarine cables. But sometimes you have to forget about conventional wisdom and think outside the box–and you need to do this at the time that the optical engine chips are designed because you can’t change basic functions once they are burned into an ASIC. But by using these techniques, it’s possible to extend the economic life of all types of submarine cables around the world.
Geoff Bennett is the director of solutions and technology at Infinera.
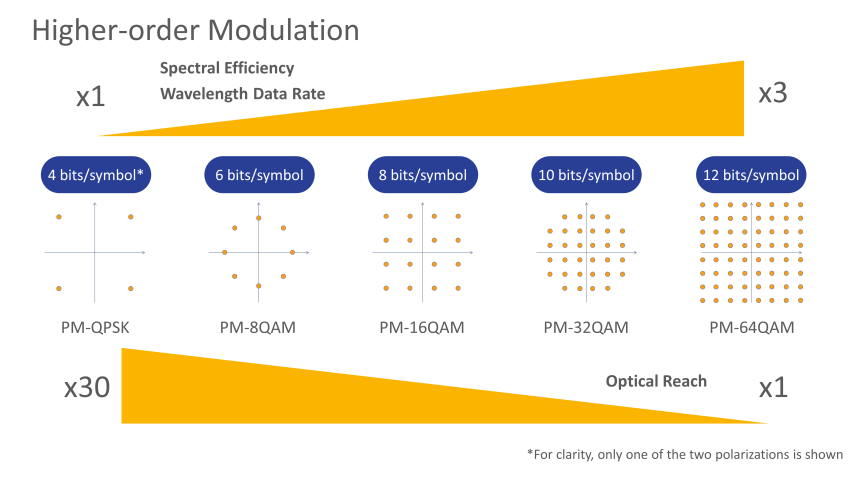
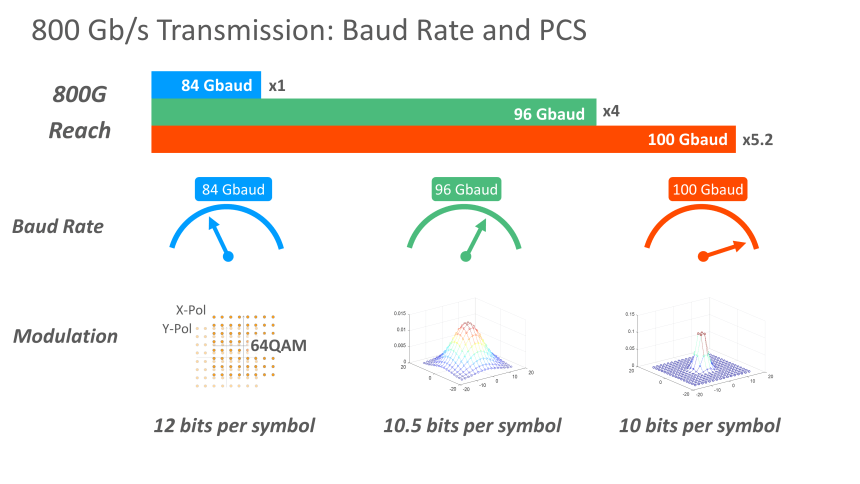
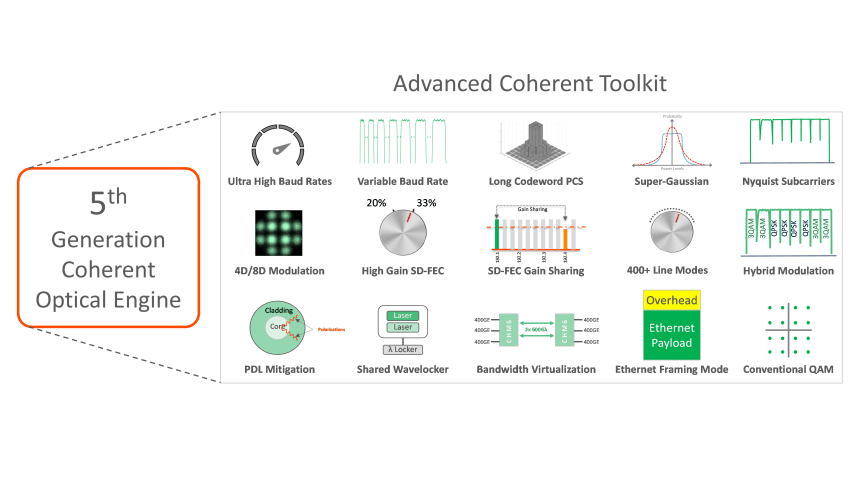